Orchids are fascinating plants, and they are particularly known for allowing a lot of crossing between species. So much that there is a special system for naming hybrid orchids: greges.
A grex (plural “greges”) is a concept that applies only to orchids, and that replaces the concept of hybrid species, which is used otherwise in botany.
First a small detour about hybrid species, and how they are used. In nature, plants belong to species. So for instance, you may have at home some houseplant from the species Kalanchoe daigremontiana, which is also called “Mother of Thousands”.
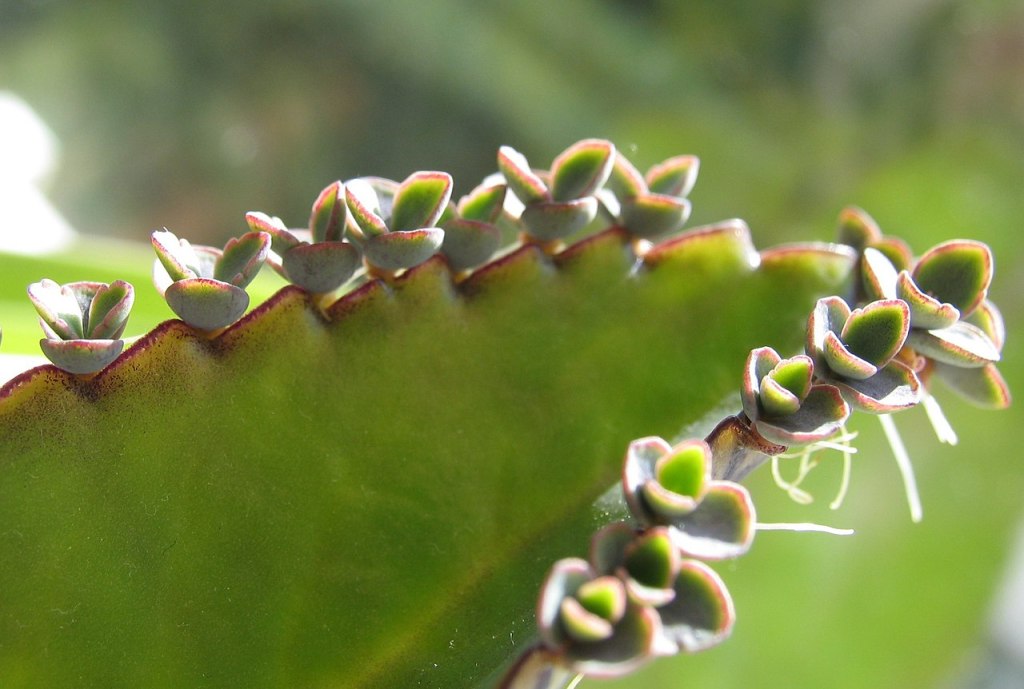
Now, this is a very beautiful plant which produces lots of little baby plants on the margin of the leaves, which tend to fall off and produces many more plants, explaining the common name. If you like this species, you may look for similar plants and come across Kalanchoe delagoensis, which has thinner leaves, but just as much little baby plants on them, and is therefore nicknamed “Mother of Millions”. In this case both “Kalanchoe daigremontiana” and “Kalanchoe delagoensis” are species and their names consist of the genus name (“Kalanchoe” for both) followed by a second word called the epithet. Together they form the species name.
If you’ve had kalanchoes than you know that they make beautiful little flowers, often in red. In fact, if you have both species, you may be able to cross-pollinate them to give a hybrid. This hybrid is called Kalanchoe houghtonii. This is not a species, but a hybrid species. Hybrid species are also called nothospecies. The important part is that species and nothospecies have the same naming conventions: A genus name followed by an Latin epithet, written in lowercase and usually in italics. We can write the situation down in a formula:
Kalanchoe houghtonii = Kalanchoe daigremontiana × Kalanchoe delagoensis
In this formula, the “×” is like a mathematical operation that takes two things (species or nothospecies) and returns a new thing (a nothospecies). This operation can be written in an abbreviated form as
Kalanchoe houghtonii = Kalanchoe daigremontiana × delagoensis
but that is purely a typographical detail that doesn’t change the meaning of the formula. In the following, I will avoid this type of shortcut.
When writing the name of a nothospecies, it is allowed to include a “×” sign before the epithet, as in “Kalanchoe ×houghtonii“, but that sign is optional, and in any case, for many species we are not certain that they are not hybrids of other species, so for most practical purposes, species and nothospecies can be considered the same thing. What should be noted is that the “×” sign is used in two different ways: As a binary operator that takes two (notho)species and returns a species, and as a prefix to denote that a name is a nothospecies. What may be even more confusing is that some people put a space between the “×” and the epithet, which gives “Kalanchoe × houghtonii”. To avoid confusion, we will never use “×” as a prefix – “×” will from here on always denote a binary operation.
Now, how is the situation for orchids? Well for botanists there is no difference, because botanists also use nothospecies to name hybrid orchids, and exactly the same rules apply. But botanists are only interested in hybrids that appear in nature. As for the thousands (or millions?) of hybrid orchids that are created in cultivation, there is a different naming system: the grex. An example is
Brassavola Little Stars = Brassavola nodosa × Brassavola subulifolia
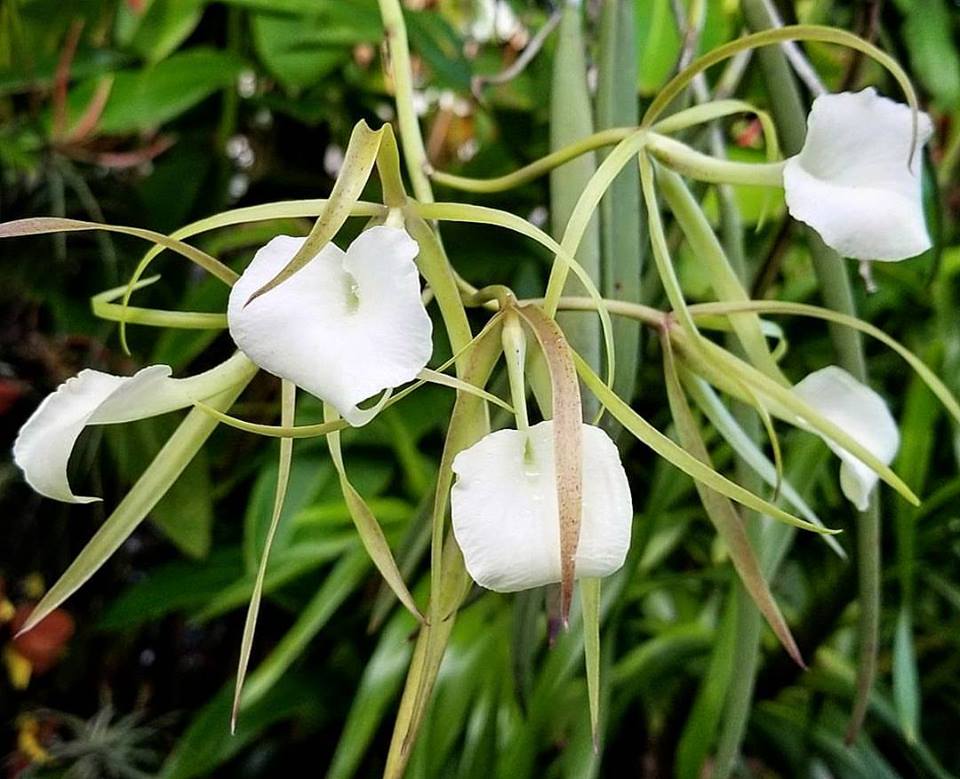
The first thing to notice is that the epithet is not in Latin. In this case it is in English, but sometimes it’s in French like in “Paphiopedilum Ma Folie” or just the name of a person like in “Papilionanda Mimi Palmer”. Also, the epithet can consist of multiple words, and the words are capitalized. Also, italics are not used. But if those were the only differences, I would not have written this blog article. The real interesting part are the rules of naming, which I have summarized in this table:
Nothospecies (any plant) | Greges (only orchids) | |
Idempotence | A × A = A | A × A = A |
Commutativity | A × B = B × A | A × B = B × A |
Associativity | (A × B) × C = A × (B × C) | ––––––– |
This requires some explanations. Let’s start with the rule “A × A = A”. In this notation each letter like A stands for a species, a nothospecies or grex. What this formula says is that if you cross a plant of type A with a plant of type A, you get another plant of type A. You cannot create a hybrid by starting with just one species. This is true for both nothospecies and greges, even if A is already a hybrid. Crossing a plant from nothospecies A with another plant from nothospecies A always gives a plant from nothospecies A. And the same is true for any grex A. The mathematical term for this rule of calculation is “idempotence”. Most mathematical operations we come across in daily life are not idempotent. “5 × 5 = 5” is not a true statement in mathematics, but
Kalanchoe daigremontiana × Kalanchoe daigremontiana = Kalanchoe daigremontiana
is a true statement in botany.
On to the second property. A × B = B × A. This means that it doesn’t matter whether A or B is the seed parent or the pollen parent. When two plants are crossed, one parent is the female parent (the “seed parent”) and one is the male parent (the “pollen parent”). In whatever way we cross A and B, we get the same result. Note that we have not even specified whether we write the seed parent or the pollen parent on the left or right of the sign “×”, but since our “×” always have the property of commutativity, it doesn’t matter. This rule works the same way for nothospecies as it does for greges. To give an example with greges this time, we can write
Brassavola nodosa × Brassavola subulifolia = Brassavola subulifolia × Brassavola nodosa
Both sides of this equation result in “Brassavola Little Stars”, and the equation is thus true.
For the third rule, we will now learn that nothospecies and greges work differently. Let’s look at nothospecies first. The rule
(A × B) × C = A × (B × C)
states that if we start with three types (A, B and C) and cross them, it does not matter which we cross first. We can cross A and B first (as written on the left side of the equal sign) or B and C first (as written on the right), the result is the same. In fact, we could even cross A and C first. How do I know that? By applying the rules of commutativity and associativity:
(A × B) × C = (B × A) × C = B × (A × C)
In this calculation, we first applied the rule of commutativity to “A × B” inside the parentheses, and then applied associativity to the result.
What is nice about the rule of associativity is that it allows us to omit the parentheses. “A × B × C” is a perfectly well-defined expression.
Imagine now that we have three species A, B and C. Which nothospecies can we construct from it? Let’s apply the operator “×” and define some new hybrid names:
D = A × B
E = B × C
F = A × C
G = A × B × C
Which other nothospecies can we define? A × A maybe? No, that’s the same as A. A × B × A maybe? No, that’s the same as A × B. You can convince yourself of that by first applying commutativity to get B × A × A, and then simplifying the expression using idempotence.
In fact, there are no more nothospecies we can define. Based on three species, we can only define four nothospecies.
As a little quiz: Given n species, how many nothospecies can we define? The answer is 2^n − n − 1. That’s because each nothospecies corresponds to one subset of the given set of species, which has n elements. There are 2^n subsets possible, but we must exclude the n given species (corresponding to sets with one element), and the set with zero elements (which doesn’t correspond to anything).
Mathematically, this all means that given a set of species S, the set S along with all nothospecies that can be derived from it forms the free semilattice generated by S. Don’t worry if “free semilattice” seems very complicated. It makes sense mathematically, but it would be too long to explain here.
Now let’s go over to the world or hybrid orchids and their grex names. Let’s start with three species A, B and C. As before we can define D = A × B, E = B× C and F = A × C. We can also define G = (A × B) × C, being careful to use parentheses, because “×” is not associative for greges. Which means that H = A × (B × C) is another grex.
Also, we can now define I = (A × B) × A. Since we don’t have associativity available, this does not simplify to A × B as was the case with nothospecies.
We can also define J = ((A × B) × A) × B, H = (((A × B) × A) × B) × A, and so on. And even something like ((((B × A) × A) × A) × A) × A cannot be simplified. What this means is that even starting with just two orchid species, we can in theory define an infinite number of new greges.
To give a an example of this, consider the following orchid greges:
B. Solstice Star = B. subulifolia × (B. nodosa × B. cucullata)
B. Little Yaki = (B. subulifolia × B. nodosa) × B. cucullata
I’ve abbreviated “Brassavola” as “B.” to make the formulas shorter.
What we can observe here is that Brassavola Solstice Star and Brassavola Little Yaki are both hybrids of the same three Brassavola species, but in a different order. And since they are greges and not nothospecies, they have different names.
Mathematically, a set of orchid species together with the (infinite) set of possible greges would form something like a “free non-associative semilattice”, but I don’t think mathematicians use the expression “non-associative semilattice”. I’m sure someone can help me find the correct mathematical term.
To conclude, I’d just like to point out that since so many orchids can be hybridized, there is never a shortage of grex names, and new hybrids get defined all the time. Orchid cultivators give all possible names to new hybrids, sometimes to honour someone, but sometimes just because a word is funny.
Just search for a funny word like “banana”, and you’ll find such gems as
Paphiopedilum Top Banana, Bulbophyllum Anna Banana, Calanthe Mana Banana, Lycaste Banana Pine, Oncidoglossum Banana Boat, Rhynchobrassoleya Banannarama, Vanda Habanananda, Zootrophion Pink Banana, Bulbophyllum Banana Split
and many many more.
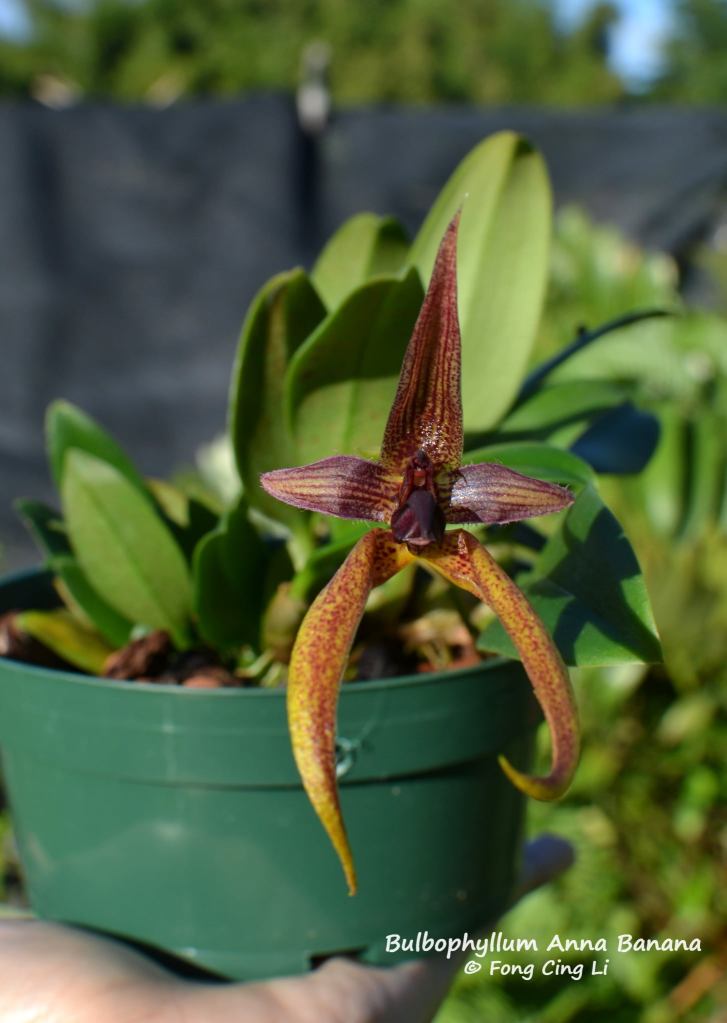